Personalized brain mapping incorporates a methodology which innately promotes its use in surgical settings. First and foremost, the ability to visualize and freely segment named brain networks and associated tract bundles is of great importance in planning surgical trajectories. Additionally however, personalized brain mapping brings into the hands of a surgeon: 1) a greater clarity of brain network involvement in surgical pathology pre-operatively, 2) the ability to structurally inform maximal safe resection intraoperatively, and most importantly, 3) enhanced predictions of potential postoperative consequences, generating a complete informed consent for patients.
Brain network involvement
Pre-operative assessment of a patient’s brain networks gives surgeons a thorough understanding of how pathology has already impacted functional brain networks. Damage to these networks can cause deficits in the functions in which they underpin or manifest entirely new cognitive or neuropsychiatric disturbances.
Taking for example the three main cognitive brain networks – the Central Executive (CEN), Default Mode (DMN), and Salience Network (SN). The CEN drives goal-directed behavior, and conversely, the DMN activates during passive states of mind and at rest17-19. The balance of switching between these two networks during daily life is mediated by the SN20. Unsurprisingly, damage to or disconnection of the tracts between these major networks can cause impairments to higher-order cognitive abilities and cause symptoms associated with schizophrenia, depression, and anxiety21-23. The use of personalized brain mapping preoperatively informs neurosurgeons as to the precise location and involvement of these networks, among others, in their patient’s pathology, allowing them to plan accordingly to avoid and navigate these networks during surgery24–26.
Maximal safe resection
The precise anatomical visualizations produced by personalized brain mapping empower neurosurgeons seeking a safe maximal resection. As they are informed by tracts connecting functional gray matter areas, not only can a surgeon use personalized brain mapping to see where a tumor site is entangled with functioning brain tissue, but also if adjacent gray matter has already become compromised by a tumor’s presence prior to surgery. This information not only informs surgeons which still-functioning tracts and networks to avoid in their approach, but also which already compromised tissue can be safely resected without leading to worse post-operative symptoms27-29.
Better patient consent
Since the 1940’s, consent laws have been in place to ensure that patients are made fully aware of the potential risk-factors of intracranial procedures30. By displaying the location of brain networks and their connections relative to structural damage, personalized brain mapping enables a neurosurgeon to directly demonstrate to their patient where and why performing the elected surgical strategy could result in postsurgical deficits1,4. Instances where a patient has awoken from surgery with an unexplained deficit have shown to result from components of core brain networks being damaged in the surgical approach31-35. What is of paramount importance in cases such as these is that patients are made aware of how their deficits relate to changes in their brain’s structure and function - which is made possible by personalized brain mapping36,37.
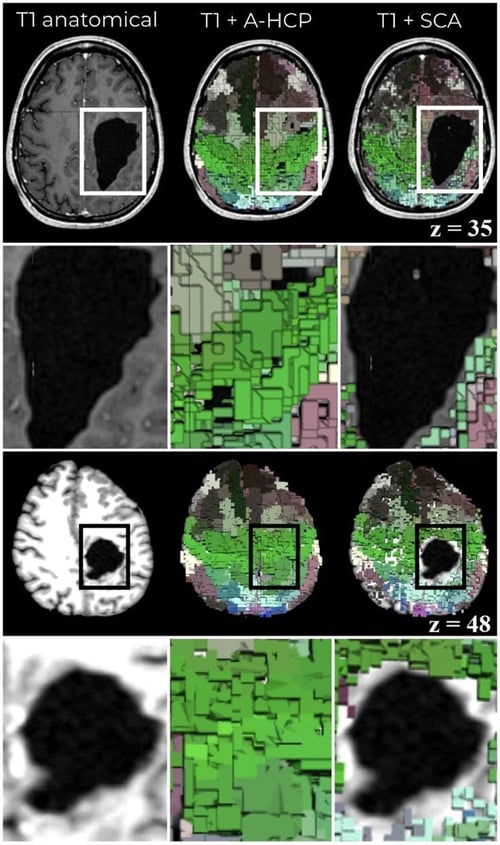
Figure 3. Personalized brain mapping resolving functional areas around structural pathology. Left column: a T1-weighted image showing the location and extent of structural damage. Middle column: a typical brain atlas overlayed on the T1 image, demonstrating an inability to account for structural damage. Right column: The Structural Connectivity Atlas (SCA) personalized brain mapping technique, showing that brain regions are mapped in accordance and surrounding structural damage.